To mark the occasion of Nature Chemistry turning 10 years old, we asked scientists working in different areas of chemistry to tell us what they thought the most exciting, interesting or challenging aspects related to the development of their main field of research will be — here is what they said.
Whether the notion was first articulated by a famous physicist, beloved baseball player or simply someone else, there is no doubt that predictions can be difficult, especially about the future. Nevertheless, another famous quote (of slightly more certain origin) suggests that perhaps the best way to predict the future is to actually invent it yourself. We are confident, therefore, that the collective vision presented below in this article — from researchers who are actively pushing forward the frontiers of chemistry and helping invent its future — offers a credible blueprint for the challenges our subject will face, and how it will evolve in the coming years.
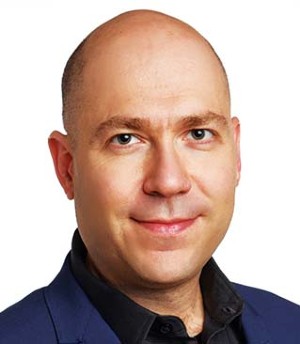
Alán Aspuru-Guzik: One of the ultimate goals for computational chemists is to achieve inverse design, which means going from desired properties to a stable and synthesizable molecule. Using artificial intelligence (AI) to drive generative models that ‘dream up’ new candidate molecules shows promise to make this a reality. To close the discovery loop, the integration of AI and robotics into both synthesis and characterization is one of my areas of focus. In addition, the increasing power of quantum computers alongside advances in quantum algorithms for the simulation of molecules suggests that they will soon be competitive with current classical computers.
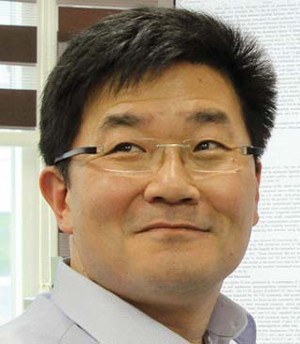
Mu-Hyun Baik: As computer models of chemical reactions become more realistic, we must learn how to use them to enable greater creativity and innovation. Machine learning and artificial intelligence will help, but human learning and natural intelligence will have to do the lion’s share of the job for at least the next 20 years. Can we use computer models to design catalysts for challenging reactions from scratch? Can we come up with real and disruptive innovations that defy common chemical sense? I think this is entirely possible — we just have to do it. Unfortunately, it’s easier said than done.
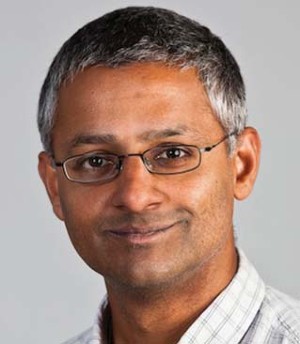
Shankar Balasubramanian:The primary sequence of DNA can now be rapidly determined for human genomes, enabling DNA sequencing to occur at a larger scale. The next decade will establish more fully how, and in what situations, this information can explain who we are or help improve disease management. On a more molecular level, it is becoming clear that the structure and the covalent chemistry of DNA are dynamic in living systems. Understanding how, why, and when DNA undergoes such changes will reveal molecular mechanisms — other than Watson–Crick base pairing — by which DNA may store and transmit instructions. Nature has been ‘exploring’ DNA nanoscience much longer than we have in our laboratories.
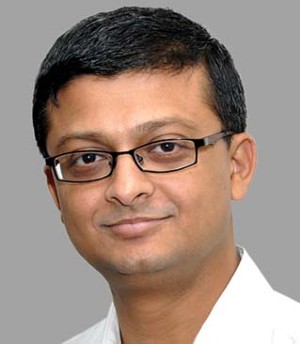
Rahul Banerjee: The next generation of porous crystalline polymers or covalent–organic frameworks should overcome the existing limitations of dynamic covalent chemistry (DCC). The diversity of covalent bonds allows materials to be produced with fascinating properties because of the varied chemical properties of the individual organic building blocks and the directions of their linkages. Once we push the synthesis of porous crystalline polymers beyond the boundaries of DCC, these materials will bring structural diversity while unleashing exciting new properties, and there will be new opportunities for the polymer industry to investigate these materials for commercial applications. Another interesting advance could be the design of flexible and responsive crystalline covalent networks.
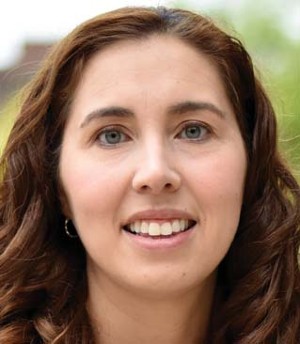
Suzanne Bart: Actinide chemists are at the forefront of synthetic inorganic chemistry, and get to push the boundaries of the periodic table daily. The surprising reaction products that the 5f-elements deliver constantly challenge actinide scientists to be open-minded and think creatively about chemical behaviour. Recent advances in equipment and computational techniques have mitigated difficulties with handling radioactive materials, opening up this research area for further exploration. Being a part of the community that is helping to establish basic chemical properties and uncovering fundamental reactivity for these elements through inorganic synthesis is an exciting endeavour.
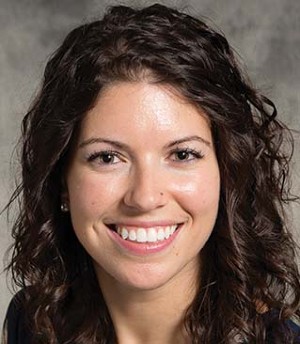
Nadine Borduas-Dedekind: Thanks to advances in in situ and real-time analysis, particularly in mass spectrometry, atmospheric chemists now have unprecedented information about the molecular composition of gases and particles in today’s atmosphere. Going forward in climate research, the task is to quantify the human fingerprint in our changing climate. One promising, yet challenging, approach is to measure and model the atmospheric composition in remote regions. In air quality research, the goal is to link our exposure to atmospheric constituents to adverse effects on health. I am excited about the innovations atmospheric chemists are bringing to indoor air quality, by quantifying reactive species, and by studying the fate of short-lived air pollutants and their impact on human health.
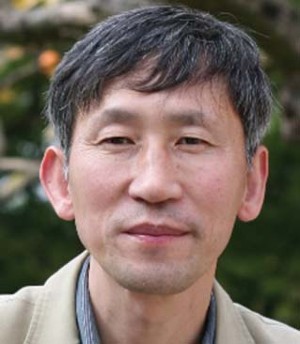
Sukbok Chang: One of the most exciting research topics in synthetic chemistry is to gain a better fundamental mechanistic understanding to develop catalytic systems that enable kinetically and thermodynamically unfavourable transformations to occur. In particular, direct C–H bond functionalization of readily available feedstocks — simple hydrocarbons, for example — to afford value-added compounds would be highly desirable. One immediate target methodology is the dehydrogenative cross-coupling of hydrocarbons using a C–H bond activation strategy and environmentally benign oxidation tools. Reactivity and selectivity can be tuned by designing catalyst systems that can bypass the need to pre-functionalize starting materials and also avoid hazardous byproducts.
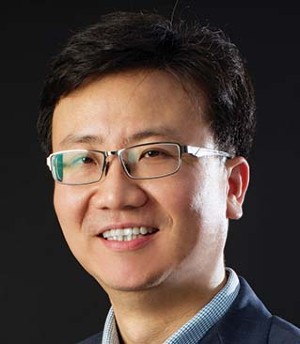
Peng Chen: A method to amplify proteins in a manner analogous to the polymerase chain reaction for DNA and RNA molecules would open many exciting frontiers, ranging from single-molecule protein sequencing to single-cell proteomics. The more complex nature of proteins, however, in particular the nature of the 20 distinct amino acid side-chains, presents a formidable challenge to fulfil this dream. Nevertheless, the development of a collection of efficient, orthogonal chemical or enzymatic reactions that can selectively convert different types of amino acids into amplifiable and distinguishable signals, in conjunction with innovative data processing methods, may ultimately produce a breakthrough technique for turning proteins into amplifiable molecules.
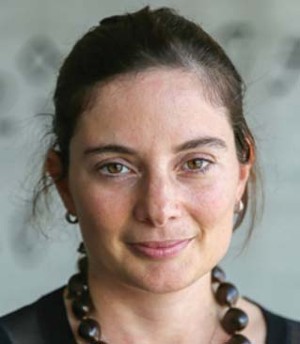
Clemence Corminboeuf:Computational chemistry is currently undergoing several fundamental changes with advances in, for example, machine learning and big data analysis, GPU (graphics processing unit)-accelerated software and quantum chemistry on quantum computers. In particular, the machine learning of quantum chemical properties is booming and will likely lead to an acceleration in quantum chemical computations, providing new frameworks and protocols to quickly and efficiently screen and discover novel molecules and materials. While traditional approaches will remain, the increased use of machine learning will transform the nature, scale and complexity of problems that can be tackled computationally. Some remaining cross-community limitations will likely be overcome by creating national and international synergic networks that unite diverse scientific communities.
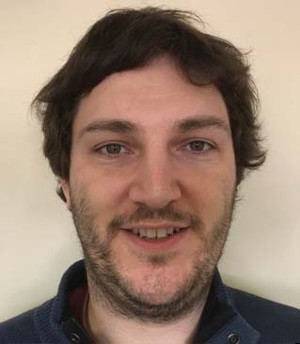
François-Xavier Coudert:Machine learning is already changing computational chemistry, integrating at various levels in methodologies including interatomic potentials, density functional theory (DFT) functionals and structure–property relationships. The biggest challenge is in scaling up these efforts, with large datasets from every team’s latest research following open-science principles: fully accessible, associated with well-defined metadata and using interoperable formats. Not only can this improve reproducibility and speed up the pace of discoveries, but it will enable data mining in a way that’s never been possible before in chemistry: every calculation, every experiment — whether the synthesis was successful or not — all at your fingertips to create the next breakthrough or wonder material!
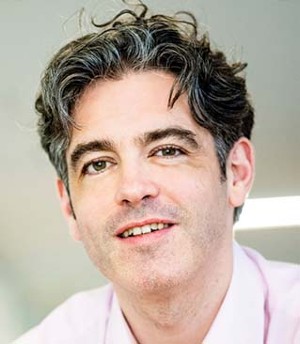
Leroy Cronin: In the search to create a truly bottom-up artificial life-form that does not rely on information from current biology or technology, the development of chemical systems that can process their own information (free from an observer) is crucial. Right now, such systems are controlled by a human chemist or molecular-level biological machinery, but what if it was possible to produce a system that can create itself and its own information, making chemical messengers to inform its structure or function? The emergence of self-programming chemical systems would be an important landmark in the development of autonomous artificial assemblies created from scratch in a ‘from sand to cells’ approach.
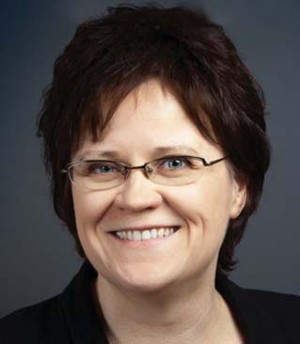
Cathleen Crudden: Bringing molecular-level synthetic approaches and analytical techniques to materials chemistry is extraordinarily demanding but also incredibly interesting. We are approaching this challenge in my group by employing N-heterocyclic carbenes (NHCs) as ligands for metallic superatom clusters. The advantage of the carbene is that the metal–carbon bond provides a beautiful handle for NMR analysis, enabling direct characterization of the ligand–nanocluster bond. The clean molecular chemistry of NHCs enables the development of logical synthetic routes for nanocluster synthesis, which we hope will be applicable to wide classes of nanomaterials.
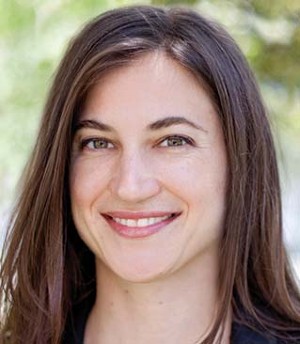
Tanja Cuk: The most exciting aspect of investigating chemical transformations at surfaces is the opportunity to reveal the true dynamics of a catalytic cycle. The goal is to not only capture catalytic intermediates in time, but monitor how they move along the surface, interact with each other, create the next intermediate, and then finally the product’s bonds. To establish this mechanistic understanding, we need advanced spectroscopy to be applied to catalytic reactions in situ with high temporal resolution over many orders of magnitude. In short, if we could identify how the transition states that enable continual and catalytic product evolution are reached, it would move the field of heterogeneous catalysis into profoundly new territory.
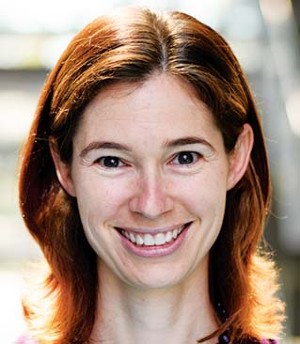
Abigail G. Doyle: Chemical reactions and molecular structure are complex, high-dimensional spaces. Synthetic chemists have extensive knowledge and intuition in this domain, yet the discovery and optimization of chemical reactions still consumes significant time and material resources while making limited use of data. Invention of tools at the nexus of machine learning and chemistry will augment current practices, accelerating the pace of molecular discovery essential to many outstanding problems of societal concern. Progress will require a diverse group of scientists and engineers to invent new approaches to data collection and curation, the description of chemical space, and predictive and interpretable algorithms tailored to chemical problems.
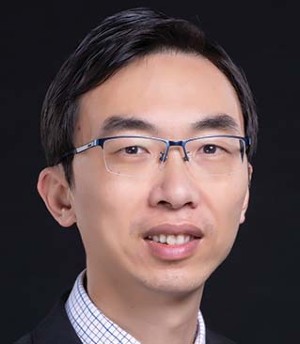
Chunhai Fan: An exciting frontier is to understand how artificially designed nucleic acids assemble and function inside living cells and in animals. Devising new tools to manipulate the assembly of nucleic acids — both natural and synthetic — within live cells would revolutionize nucleic acid chemistry, and in turn enable advances in the design and fabrication of nanoscale theranostics for precision medicine. Thinking further into the future, another direction offering promising applications is to explore the development of artificially intelligent DNA or RNA robots that can function on demand in animals and in human beings.
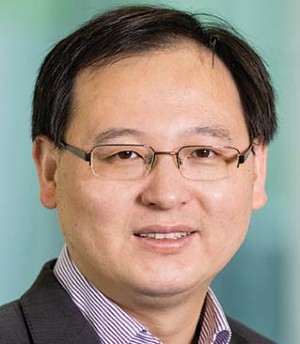
Xinliang Feng: A major goal of synthetic chemistry is to create materials that can help tackle the current societal challenges, through, for example, the increased use of sustainable energy, smart manufacturing or health informatics. Novel condensed matters with intriguing physical or chemical properties for the transport of electrons, spins, ions and phonons have long been attractive synthetic targets. Achieving these materials will require the development of new synthetic methodologies and strategies. This in itself will necessitate creative thinking, in order to design controlled chemical syntheses of solid-state materials with complex structures and tailor-made properties at the atomic and molecular level.
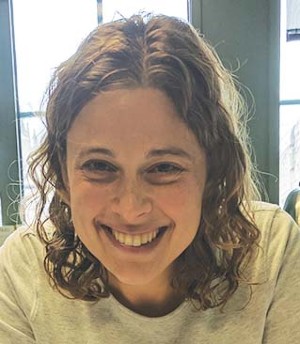
Danna Freedman: Quantum information science promises to transform our scientific landscape. Chemically derived qubits are particularly attractive in the subfield of quantum sensing, in which the quantum properties of a species are harnessed to detect extremely small signals such as minor variations in temperature, magnetism or electric fields. Over the next decades this area may seed advances such as the single-molecule sensing of protons and electronic spins, leading, for example, to single-molecule NMR that will enable detection of the small proportion of enzymes that are in a specific state of interest (such as catalytic intermediates). Progressing from sensing ensembles to sensing single molecules will impact our understanding of reaction mechanisms, biological systems, and exotic materials.
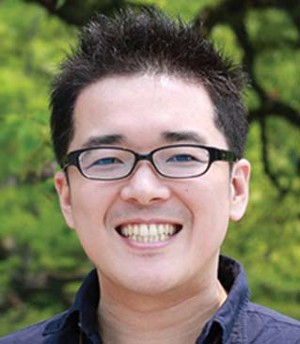
Shuhei Furukawa: An exciting frontier in metal–organic frameworks (MOFs) is their reconfigurable microporosity in response to mechanical stress. We are now just beginning to understand the mechanisms for flexibility in MOFs, and to design materials with multi-well potential energy landscapes. Gaining further insight into how to translate macroscopic force into molecular motion in hierarchical structures would facilitate the tuning of microporosity. The key to achieving such ‘mechano-pores’ is to reconcile long-range cooperativity and local structural freedom; I believe that the recent trends of controlling defects and disorder in frameworks, and of fabricating soft matters such as gels, will lead to this advance.
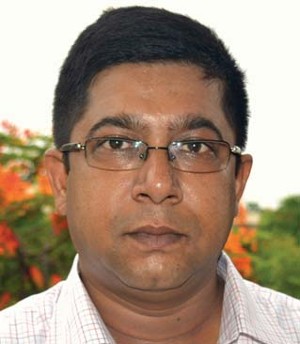
Suhrit Ghosh: Supramolecular polymers, even though they have an internal order, lack macroscopic structural regularity. Emerging reports suggest that temporarily trapping the monomer in a dormant state enables new possibilities in controlled supramolecular chain-growth polymerization using a suitable initiator. In the recent past, it has been possible to synthesize supramolecular polymers with remarkably low dispersity and a predictable degree of polymerization. Supramolecular copolymerization of multiple building blocks in sequential fashion is now within reach, similar to covalent block copolymers. This fundamental advance opens up new opportunities for realizing complex molecular assemblies with structural precision on different length scales, such as those ubiquitous in biological systems.
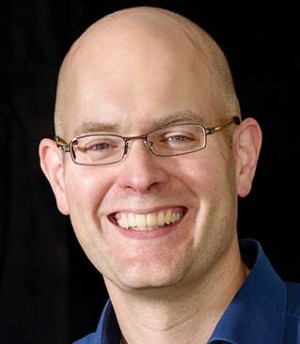
Frank Glorius: This is a golden era of catalysis, and with a plethora of catalytic technologies and ever-improving analytical tools being developed, the most exciting and important challenges await. I am especially excited about three areas of development. First, heterogeneous catalysis — greater molecular understanding is required to enable the design of new catalysts for more efficient industrial processes. Second, new strategies and improved catalysts for the late-stage functionalization of complex molecules (for example, by the use of C–H activation). Finally, I believe the discovery of new reactions using information-based strategies, such as smart screening or artificial intelligence and machine learning, will dramatically change the field of catalysis and chemistry.
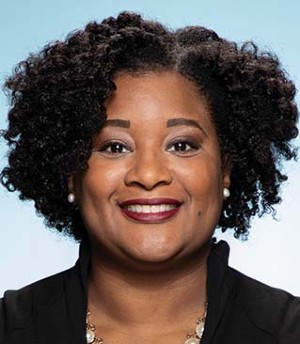
Malika Jeffries-EL: In recent years, organic semiconductors have evolved beyond fundamental academic research, and are now used in numerous commercial applications. Despite their potential, new materials that have ideal properties and can be made using simple synthetic protocols are needed for ‘plastic electronics’ to be feasible. The performance of an organic semiconductor relies on the interplay between its structure and opto-electronic properties — both of which are dependent on the optimization of many interrelated variables such as energy levels, band gap and charge transport. Thus, new computational tools, synthetic protocols and the synergistic collaboration of organic chemists, theorists, physicists, materials scientists and electrical engineers are needed solve these challenges.
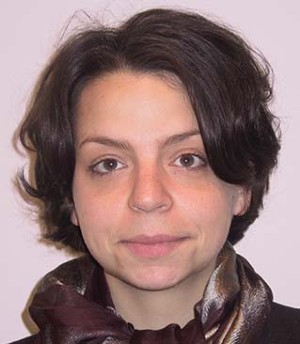
Nathalie Katsonis: The most exciting challenge in my research field is the unravelling of the rules of molecular motion, because they will help us set inanimate matter in motion. We still don’t know how abiotic chemistry could have ever transitioned into the directional motion that is an essential feature of life, but I feel strongly that artificial molecular machines, on which I work, will provide this insight. En route, I look forward to unrivalled man-made molecular machineries that work together and generate movement in an auto-adaptive matter, using feedback loops and non-linear responses. Ultimately, this chemistry might create motile protocells that evolve collective and purposeful behaviour.
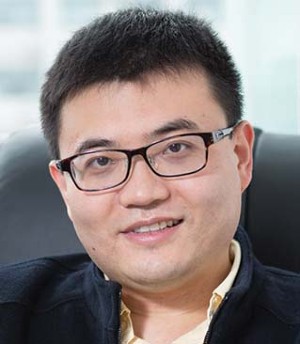
Ang Li: In the field of natural product synthesis, designing synthetic routes with the help of computers may become an increasingly attractive direction. In contrast to rather ‘flat’ pharmaceuticals, stereochemically complicated natural products remain challenging targets for computational methods. Strategies based on systematic analysis of biosynthetic networks to uncover underappreciated retrosyntheses could significantly improve the practicality and versatility of computer-designed routes. In addition, the gene clusters responsible for enzymatic reactions (or reaction cascades) in natural product biosynthesis could be an advantageous tool for computers, potentially making combined chemical and enzymatic routes more common in near future.
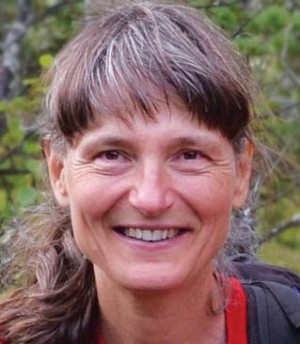
Sara Snogerup Linse:Molecular self-assembly is a fundamental process behind the formation of biological structures such as membranes, organelles, and multimeric proteins. The self-assembly of proteins and peptides is also involved in several devastating human diseases, although the exact connection between the assembly process and pathology remains to be found in many cases. Are the aggregates a cause or a consequence, or a contributing factor, among others? Can we control assembly and disassembly using modulators based on physicochemical principles? Can we learn from nature and make switchable self-assembled materials with tunable properties? To answer these questions, we need to unravel the chemical features that discriminate between beneficial and pathogenic assemblies.
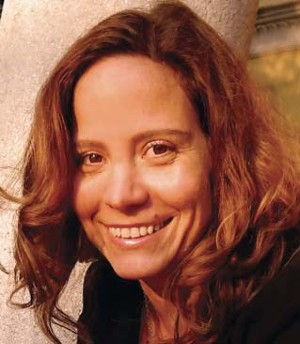
Silvia Marchesan: Chirality has fascinated chemists for nearly two centuries, and still delivers unexpected marvels at scales ranging from the sub-atomic to the galactic. Homochirality plays a significant role in nature, but the importance and potential utility of heterochiral assemblies for building functional macroscopic networks should not be underestimated. Supramolecular systems that aspire to mimic the elegant complexity of nature also need to be sustainable. A grand challenge is to decipher the design rules that will enable us to code information into heterochiral building blocks so that they can self-assemble into hierarchical and dynamic structures with defined functions, just as nature assembles the components of life as we know it.
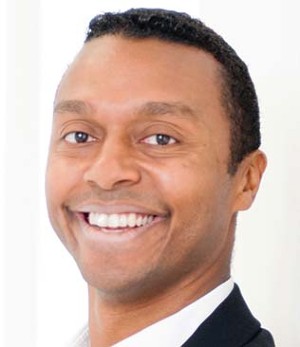
Nuno Maulide: Exciting opportunities in organic synthesis certainly include the development of tailored approaches for the manipulation of C–H bonds in organic compounds, but also the discovery of new reactions for the ‘deconstruction’ of abundant man-made compounds and biomass. Indeed, the field of organic chemistry has undoubtedly acquired a taste for C–C bond-forming reactions, but the advent of equally powerful C–C bond cleavage processes has lagged behind in comparison. The extension of finely crafted chemical processes to the realm of smart materials and adaptable organic molecules is also likely to be a game-changing endeavour.
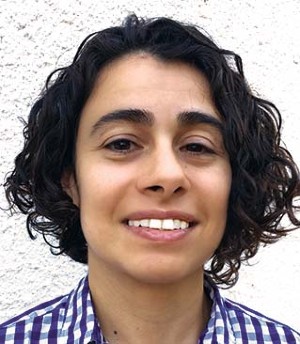
Anat Milo: In this age of automation, synthetic chemistry is increasingly streamlined, and molecular design is augmented by algorithms that juggle numerous features we cannot easily capture in our minds: the future of synthesis is bright and intriguing. A major challenge will be to integrate our understanding of chemistry into 21st-century technologies, rather than simply following the latest trends. In addition to perfecting the task of making molecules, our role as chemists is to realize their potential, unravel mechanisms and devise a sustainable future using all of the methods in our toolbox — old ones, new ones, and those that remain to be discovered.
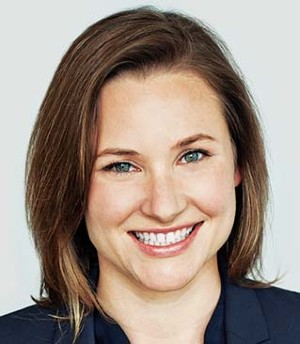
Alison R. H. Narayan: With exponential growth in the number of natural protein sequences available and the ability to engineer proteins, the potential of biocatalysis is at an all-time high. The impact of biocatalysis on synthetic chemistry in the next decade is limited only by the creativity of chemists in co-opting nature’s platforms for catalysis, and the willingness to put enzymes in our flasks. Understanding the mechanisms that gate catalysis and dictate selectivity in these systems will enable new reaction manifolds and offer solutions to challenges underserved by traditional methods.
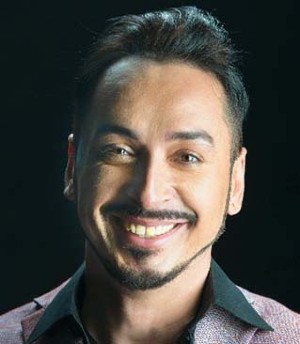
Panče Naumov: Molecular crystals are a distinct class of materials that combine structural control inherent to their anisotropic nature with rapid energy transfer, mechanical compliance and softness comparable to mesophasic materials. A plethora of publications describe their structure, yet a lingering question remains — how applicable are they really? The answer is rooted in the engineering principles of design and optimization, a language that is hardly legible to chemists. The focus of this research must shift from structure, to property, to function, and unless performance indices are established, the exciting properties of molecular crystals will remain between hype and promises.
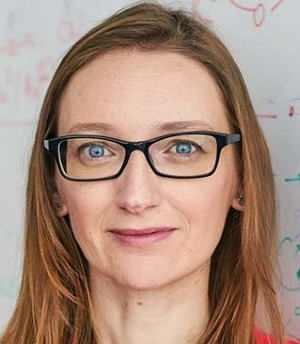
Cristina Nevado: Challenges posed by the increasing demands of a growing population on a shrinking pool of natural resources will need solutions grounded in chemistry. These include the development of highly selective, energy-efficient, environmentally benign methods to produce revolutionary new forms of matter with tailored properties, as well as the discovery of processes to efficiently transform existing feedstocks to ensure the sustainable production of new, and existing, substances. Catalysis, in all its forms, as well as a deeper mechanistic understanding of chemical processes, will play a central role to match current and future societal needs.
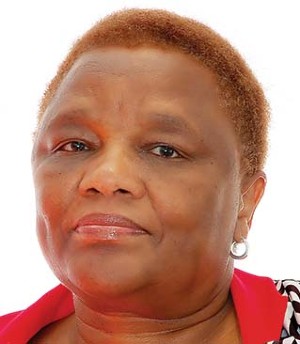
Tebello Nyokong: The resistance of microorganisms to drugs, and relentless air and water pollution represent major scientific challenges. New, ‘smart hybrid’ materials that act as photocatalysts offer exciting prospects in these critical areas. These hybrid materials combine different desirable properties in separate components, but working together they form a unique multifunctional structure. Sustainable and green, photocatalysts are a smart approach as they do not liberate any additional pollutants during water treatment, while the probability of microorganisms developing resistance to them is low. Progress in this field will rely on the development of hybrid materials which can degrade pollutants while at the same time eliminating pathogens.
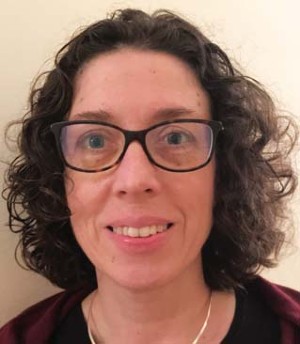
Rosa Palacin: The current landscape of battery research is really exciting because the main research directions are being driven by applications related to transport electrification and the integration of renewables. The main goals are increasing battery lifetime and enhancing energy density through the use of metal anodes, both requiring skills in electrochemistry, materials science and engineering. Machine learning and artificial intelligence have recently emerged as paths that should hopefully catalyse disruptive findings. Last, but not least, sustainability is essential: critical materials must be avoided, recycling should be promoted and the use of manufacturing processes with low CO2footprints made mandatory.
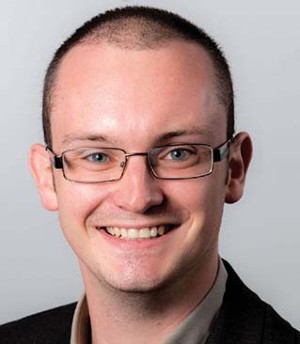
Marc Reid: Nature Chemistry’s 10th anniversary falls close in time to the 10th anniversary of Sheri Sangji’s tragic death resulting from an avoidable laboratory accident at the University of California, Los Angeles. Although there is understandable excitement about future developments in reaction-monitoring technologies, cheminformatics and programmable late-stage methylation chemistries, none of these investigations matter if they cannot be accomplished safely. None of them matter if we go to work and never come home. Therefore, the question that should most deeply challenge us is this: How can we better understand and eliminate our failings in laboratory safety?
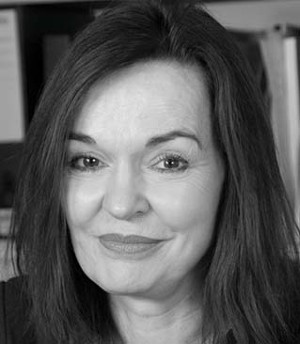
Carol Robinson: Mass spectrometry can be used to understand how membrane proteins interact with their lipid surroundings. This is challenging because most experiments require extraction of the protein assembly from the cell membrane, and this process often perturbs protein–lipid interactions. Keeping membrane proteins in their native state with their associated lipids intact, while the complex is transferred into the gas phase, is a major goal. An exciting breakthrough for us has been to release protein complexes from lipid vesicles and to eject them directly into the mass spectrometer. Future developments will involve adapting this technology to membranes from a range of different tissue types.
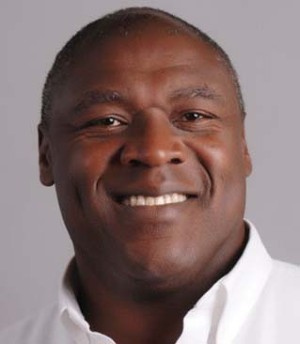
Gregory Robinson: The global challenges that confront us — such as the development of renewable energy, the eradication of disease, establishing more efficient food production processes and combating climate change — are daunting. While the solutions to these disparate problems will necessarily be multifaceted, the chemistry of earth-abundant and main-group elements will undoubtedly play a major role. Notably, the chemistry of these problems, and the chemistry of the solutions to these problems must be effectively communicated both to the general public and to governmental officials. Consequently, we must place greater emphasis on public speaking and general writing skills in the training of chemists. The future will demand both elegant chemistry and eloquent chemistry communicators.
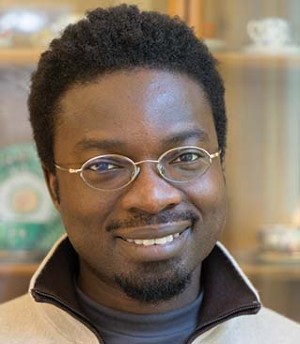
Richmond Sarpong: Organic synthesis underpins a large swathe of the pharmaceutical, materials and agrochemical industries that improve our lives. In the 21st century we should aim to master the ability to not only manipulate the periphery of organic molecules, but also precisely break and reform carbon–carbon bond frameworks of organic compounds; so-called ‘C–C activation’. Another exciting direction — made possible by advances in information and computer technology, as well as the intersection of many diverse fields such as machine learning and data science — is to use computers to accelerate the discovery of robust pathways (strategies) to prepare complex molecules. Really practical computer-assisted synthesis is very much on the horizon!
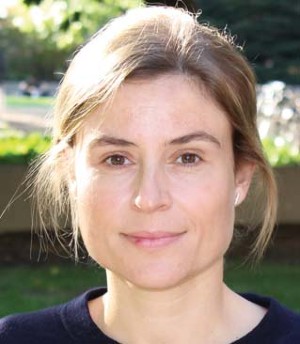
Corinna Schindler: Lewis acid-catalysed carbonyl–olefin metathesis reactions were recently developed as new methods for carbon–carbon bond formation. The most exciting aspect of these transformations is the promise they hold as alternative strategies to enable carbonyl olefinations and produce functionalized alkenes. New and more powerful Lewis acids will be necessary, however, to significantly broaden the substrate scope of catalytic carbonyl–olefin metathesis. Consequently, the development of new catalytic systems that can efficiently activate currently unreactive substrates, extending the range of products we are able to create, will be the most interesting and rewarding aspect of future research in this area.
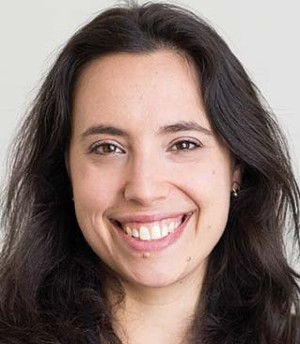
Gabriela S. Schlau-Cohen:Seeing is believing. Membrane proteins are known to manage the flow of information and material in all domains of life, but what underlies this remarkable feat remains blurry. Advances in molecular-level manipulation and measurement are sharpening our view of these proteins in both space and time. Control over the dynamics of their chemical and energetic properties — potentially even in real time — is the next frontier, where there is potential for new technologies in human health and agriculture alongside fundamental understanding of these vital and ubiquitous molecules.
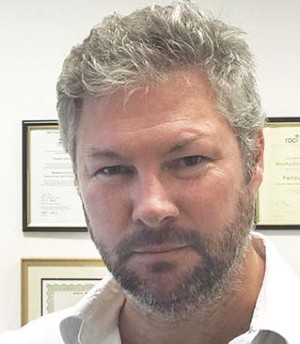
Timothy W. Schmidt: Singlet fission is now known to occur in many molecular systems, and design rules for rapid and efficient endothermic singlet fission are emerging. To properly harness this phenomenon and boost solar-energy conversion efficiencies, we must learn how to efficiently transfer molecular triplet excitons to semiconductors such as silicon, either directly or mediated by photons. The most challenging development will be discovering efficient endothermic singlet fission materials that do not degrade under sunlight.
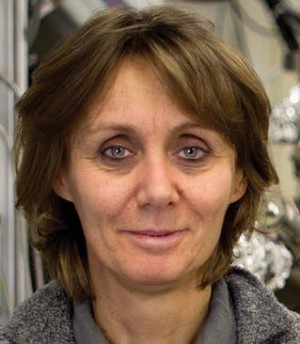
Roberta Sessoli: Better performing catalysts, less-energy demanding devices, and more efficient energy-production processes constitute the mandatory path to be followed towards a sustainable world. The spin of the electron plays a key role in all the processes that involve electron transfer, and natural evolution has optimized these processes by selecting chirality as a key component. We are just starting to understand the richness of the interplay between the spin degrees of freedom and molecular chirality. Development of new tools to investigate and control spin-dependent electron transport at the molecular scale could also open new perspectives in the emerging field of quantum technologies.
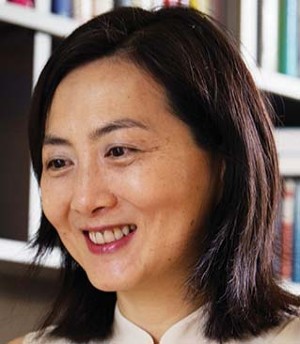
Yang Shao-Horn: An exciting frontier will be employing supramolecular chemistry to bridge biocatalysis and heterogeneous catalysis for the on-demand and sustainable production of chemicals and fuels from the reduction of water, nitrogen or carbon dioxide. To advance fundamental knowledge and applications, designing catalytic sites in three dimensions — breaking away from conventional two-dimensional catalysts — using both experiments and computation is needed, as well as controlling water structures near the catalytic sites from knowledge of ‘biological water’. Working beyond traditional disciplinary boundaries and developing new techniques for in situ and time-resolved investigations are also central to progress in this area, enabling the connection of structure with function and enhancing catalyst design.
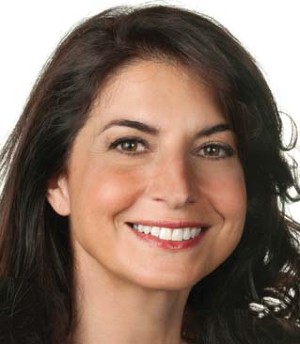
Hanadi Sleiman: Nucleic acids as therapeutics, diagnostic tools and nanostructures promise to change the landscape of medicine and materials science. The two challenges in the field are cellular delivery and large-scale synthesis. With the first siRNA therapeutic approved last year, and the first medium-scale origami syntheses now achieved, we are making excellent strides. But there is still a long way to go before we understand the interface between nucleic acid materials and the biological environment. And while many of us dream of applying the unprecedented programmability of DNA to optical, electronic, magnetic or stimuli-responsive materials, realizing this dream will require scalable syntheses and stable DNA structures.
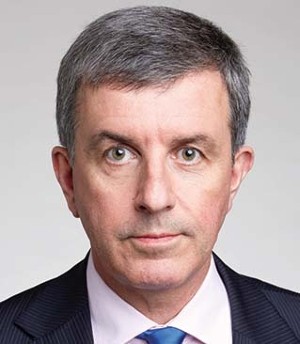
John Sutherland: In the last decade, systems chemistry approaches have hastened the discovery of prebiotically plausible routes to the amino acid, nucleotide and lipid building blocks of extant biology and not much else besides, allowing us to reject the notion that chemical selection has the power of natural selection. Refining the rough geochemical scenario suggested by the common chemistry underlying these syntheses, should, in turn, streamline the chemistry. We now seek synergistic, replicative assembly processes for RNA and peptides in lipid vesicles. We must also contemplate how energy dissipation could maintain such systems in an out-of-equilibrium state and how such processes could be sparked.
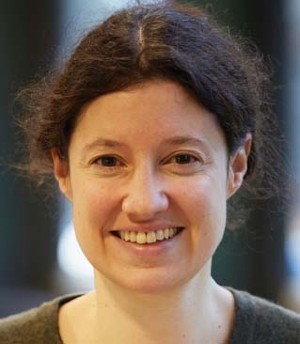
Annette Taylor: Reactions taking place in cells still provide the most effective means of producing complex chemicals, and advances in systems and synthetic biology have resulted in remarkable control over these cellular processes. An alternative approach, inspired by the goal of creating artificial life, involves the compartmentalization of networks of interacting chemicals. There is a long way to go in systems chemistry before we can control these mixtures with the same degree of robustness as biology, nevertheless, in the future there may be a shift from microbial to synthetic cell factories as we become increasingly adept in designing bioinspired reaction networks.
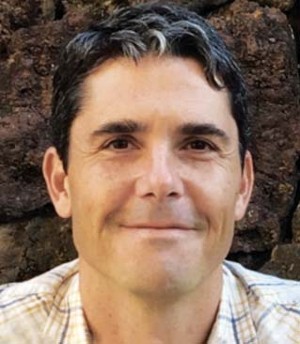
Akif Tezcan: Proteins are the ultimate building blocks for constructing sophisticated biochemical machines and dynamic materials at the nm–mm scale where inanimate becomes animate. An important frontier in molecular design will be the ability to programme the self-assembly of protein building blocks at this length scale and also their ability to predictably interact with, organize and re-organize, other forms of biological or abiological matter in a temporally and spatially controlled fashion. Along these lines, another exciting research avenue will be to engineer and evolve synthetic proteins or protein assemblies that can utilize more elements of the periodic table than contemporary living organisms.
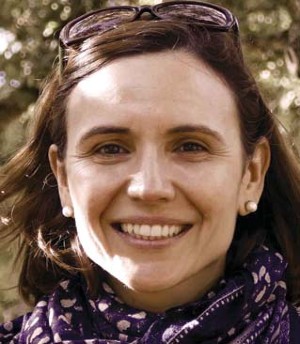
Mariola Tortosa: The development of catalytic transformations utilizing functional groups that are thought to be inert is an ongoing challenge. Methods for C–C bond activation are still in their infancy, with progress in this field changing the way scientists think about designing new molecules. Selective catalysts are needed to expand the toolbox for late-stage functionalization of highly derivatized compounds, and research in this area will have a profound effect on the acceleration of drug-discovery programmes. Selective modification of biopolymers is another exciting frontier, with the design of new methods for bio-conjugation presenting one of the most challenging opportunities for synthetic chemists.
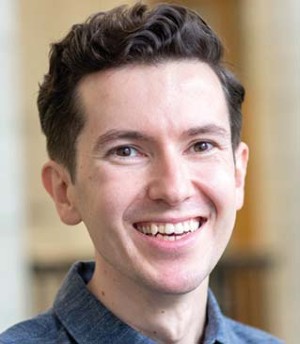
Aron Walsh: It is time for chemists to put down their lab coats and learn how to code. There are so many exciting changes happening in computational chemistry right now, from the embrace of open-source collaborative tools, the expansion of structure–property databases, to the first successes of machine learning on chemical systems. The field has completely transformed. The overlap between chemistry, mathematics, computer science and engineering is challenging but rewarding. By harnessing these tools, the future will see us exploring new chemistries and discovering materials with exotic properties far beyond what can be found in nature.
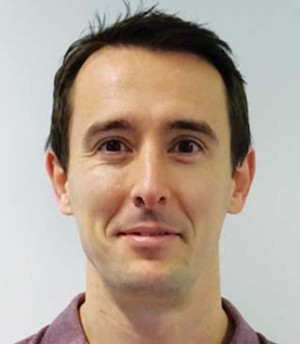
Allan J. B. Watson:Computational prediction for the a priori design of catalytic reactions will be a major theme in the ongoing development of synthetic chemistry. We are already seeing the emergence of this technology through parameterization studies of specific well-behaved catalytic systems. A significant challenge in this area will be how to rationalize and ultimately control solution dynamics. Understanding the dynamics in multi-ligand and multi-metallic systems and establishing control from the inception of a process will be transformative for the development of new catalytic reactions, facilitating novel applications and expediting chemical synthesis.
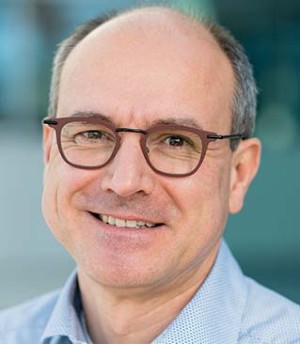
Bert M. Weckhuysen: Our society should become more sustainable, and hence we will have to think ‘circular’. Current chemical processes are designed to efficiently convert X into Y, but not yet to convert Y, after use, back in to X. Therefore, molecule or material Y will have to be designed so that we can easily recycle it and make either X or a totally new molecule or material. This way of thinking (‘looping of atoms and molecules’) will affect many fields of chemistry, including catalysis, organic chemistry and materials chemistry, because we will have to synthesize our daily goods not only to last, but also to be chemically recycled.
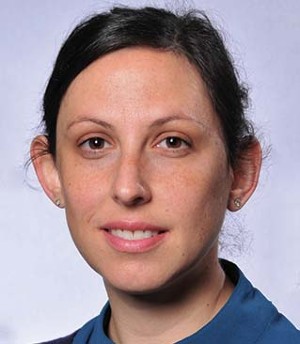
Emily Weiss: Excited state (non-thermal) chemistry is, I believe, poised to become a major force in synthetic chemistry. The reactivity and selectivity of photochemical and photocatalytic reactions will soon be controlled, not only by taking the reacting molecules out of electronic equilibrium, but also by creating new system–environment interactions to bring the entire system out of thermodynamic equilibrium. This perturbation could be achieved by coupling the system to a resonant cavity to create a new polaritonic potential surface, or to a material that responds mechanically or chemically to the reaction, such that a feedback loop amplifies the catalysis. These types of strategies come closer to natural enzymatic-type function.
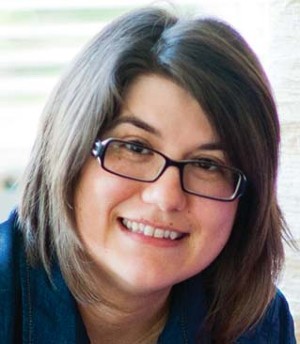
Daniela Wilson: One of the great challenges in the development of complex life-like molecular systems is to understand and design autonomous systems that can both move directionally, by harvesting different sources of energy, and also sense, communicate, interact and respond to clues from their environment, and adapt to its changes. These biomimetic motile systems are ultimately able to control their motion, directionality, speed and behaviour in response to clues from their complex biological environment. Such properties could potentially be a game changer in the biomedical field, which at the moment rely only on passive high-dose drug delivery systems.
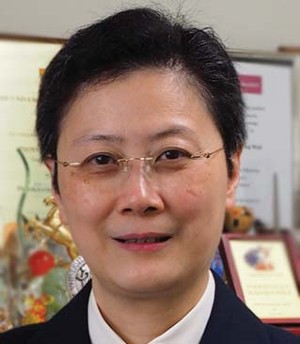
Vivian W.-W. Yam: Despite the impressive creativity and sophisticated synthetic mastery demonstrated by chemists, it has remained difficult to precisely control how molecules pack, align and self-assemble to fabricate functional molecular materials. Achieving this will rely on the manipulation of the delicate balance of various non-covalent intermolecular forces that hold the molecules together, as well as intramolecular forces that govern molecular conformation and topology. Additionally, expansion of the already diverse libraries of molecules through the generation and harnessing of their excited states — by spin-state mixing and metal-character introduction — will create virtually unlimited, exciting opportunities for breakthroughs in luminescence, photocatalysis and light-enabled technologies.
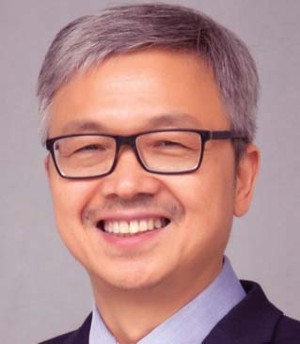
Xueming Yang: The most exciting aspect of studying chemical reaction dynamics is our ability to develop new powerful tools in both experiment and theory. New tools such as free-electron lasers in the vacuum ultraviolet and X-ray regions allow us to probe simple chemical reactions with unprecedented resolution/sensitivity and to study more complex chemical processes. Development in theory, such as more accurate DFT, enables us to investigate and understand mechanisms of more complex chemical reactions with greater accuracy. More significantly, the ever-increasing interplay between theory and experiment will surely promote the development of chemistry as a truly exact science.
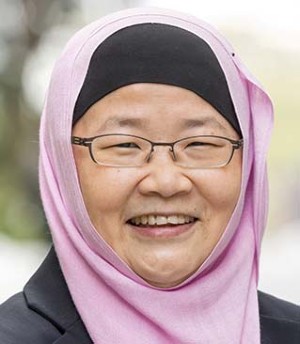
Jackie Y. Ying: Chemistry will have an increasingly broad impact in the application of new biomaterials, especially as more sophisticated systems become available and practical to use. In particular, research breakthroughs are needed in the design and synthesis of biocompatible nanocarriers that enable the controlled delivery of drugs and vaccines — preferably in an oral manner — as well as the targeted delivery of immunotherapies. New antimicrobial agents in the form of synthetic organic and inorganic materials that can bypass drug resistance are urgently needed for the control of infectious diseases in humans, animals, plants and the environment.
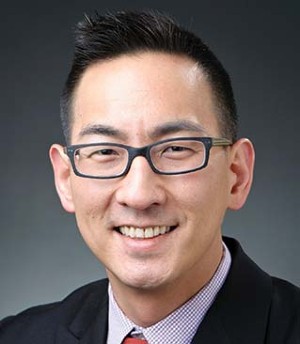
Tehshik Yoon: Enantioselective reactions require some source of chiral information. In practice, this is supplied by chiral chemical reagents, but it has also long been hypothesized that chiral physical forces might also influence the stereochemistry of reactions. Circularly polarized light (CPL) is an intrinsically chiral physical force — naturally emitted by various astronomical phenomena — and it has been proposed as a potential origin of prebiotic homochirality. To date, the only successes with CPL have been proof-of-principle experiments with quite low selectivities. A general solution to this problem, however, could profoundly impact stereoselective synthesis and provide insight into the origins of life.
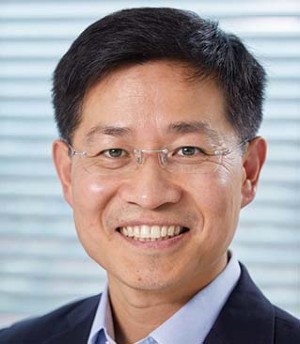
Shu-Li You: Precision synthesis is undoubtedly the premier goal in synthetic chemistry. Chemists should think how to maximize the utilization of bulk chemical feedstocks, including fossil resources and biomass, to enhance efficiency and selectivity during catalysed chemical transformations, and to promote the diversity of final products. Personally, I look forward to seeing further advances in highly efficient and selective transformations of inert chemical bonds and inert chemical systems and how the implementation of high-level theoretical calculations and artificial intelligence will fundamentally change organic chemistry.
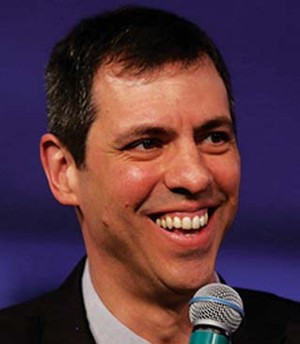
Aldo J. G. Zarbin: The growing global demand for energy has created exciting frontiers in the development of cost-effective materials that can improve the efficiency of sustainable energy technologies without damaging the environment. The search for new structures, new synthetic pathways and new processing technologies to incorporate materials into devices such as thin films will be crucial as the century wears on. Moreover, understanding the potential of emerging materials — for example, nanocarbons, 2D materials, perovskites, metal and semiconducting nanoparticles and nanocomposites — for applications in efficient solar photovoltaic conversion, facile hydrogen generation from water electrolysis, high-capacity batteries and supercapacitors and aqueous-environment operating devices represent great challenges.
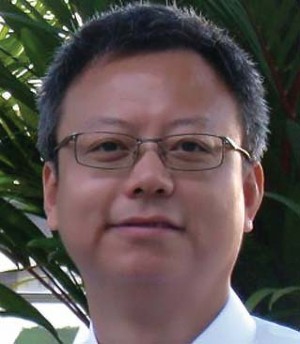
Hua Zhang: Nanomaterials have attracted considerable interest due to properties that make them promising for applications including in optics, electronics, magnetism, sensors, catalysis, clean energy and biomedicine. Many methods have been developed to prepare various kinds of nanomaterials that mainly rely on controlling the formation of composites, as well as the materials’ sizes, dimensionalities, facets, morphologies and architectures. Another route, which we pursue in our group, is a phase-engineering approach that focuses on the discovery of materials with new crystal phases — especially metastable ones — or novel amorphous structures, their large-scale controlled synthesis, and the exploration of their phase-dependent physiochemical properties for practical applications.
(원문: 여기를 클릭하세요~)